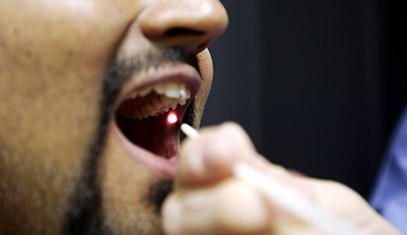
Medicine
Alzheimer’s disease, diabetes, atherosclerosis, non-toxic therapies, neurological recovery after stroke, regeneration of cardiac tissue after a heart attack
Cellular Reprogramming: A New Era in Biomedical Engineering
We are shaped not only by our genes but also by our cells' ability to execute cell type-specific gene expression programs. These programs range from differentiation into various cell types to dysregulation in disease. Although all cells in the body share an identical set of genes, their expression into proteins, starting with gene transcription, varies. As we age, most diseases are not primarily driven by gene mutations; instead, age-related alterations are mainly caused by dysregulation of transcriptional programs. For example, aging muscles lose their mechanical properties, without genetic mutation; neurons despecialize, leading to Alzheimer's disease and dementia; the heart undergoes reprogramming, contributing to cardiomyopathies; and changes in arterial cells drive atherosclerosis. Even in cancer, where gene mutations play a key role, the capacity of cells to develop new gene mutations and critical steps such as metastasis or resilience to therapies involve transcriptional changes without genetic changes. After birth, cells such as cardiac muscle and neurons lose their ability to regenerate, though some species, like zebrafish, retain this ability into adulthood. Restoring this capacity could be transformative for patients after heart attacks and strokes. How does a cell “remember” which transcriptional programs it can activate? How can this cellular transcriptional memory be harnessed? These questions have remained unanswered since the discovery of the genetic code.
Recent work at the Center for Physical Genomics at Northwestern University, using new computational, nano-imaging, and gene editing technologies, has uncovered that transcriptional information is encoded in the 3-D organization of two meters of DNA (3 billion base pairs) within each micron-sized cell nucleus. This 3-D genome geometry is an emergent and plastic reinforcement learning system, continuously evolving into thousands of nanoscopic chromatin domains, each serving as a physical element of cellular transcriptional memory. Throughout our lifespan, cell-specific chromatin domains are initiated and reinforced by stimuli experienced by the cell, competing with other domains, stored, and rewritten. Transcriptional memory determines cell function; its dysregulation leads to diseases such as Alzheimer's disease, cancer, and aging. In other words, the genome in each cell works as a computational device operating by reinforcement learning principles – similar to those used in AI systems like ChatGPT – on an “integrated circuit” of thousands of chromatin domains, each resembling a transistor function. In each cell, the number of these memory elements exceeds that in early Apple computers, and these cellular memory elements are dynamic and can evolve over time.
Deciphering the genetic code propelled modern biomedicine and led to gene-editing advances like CRISPR. Similarly, unlocking the cellular memory code will enable flexible design of cell phenotypes to tackle major health challenges of the 21st century, including Alzheimer's disease, cancer, heart attacks and strokes, tissue regeneration and rejuvenation, and aging itself. At the Center for Physical Genomics, we are developing new technologies to study and design new cellular memory codes. Recent research has shown promise in reprogramming neurons to prevent Alzheimer's disease, reprogramming stem cells to regenerate bones, programming heart muscle cells to withstand heart attacks, preventing ovarian cancer cells from developing resistance to therapies, and reverting aged cells to their younger versions. These results, once considered science fiction, are now becoming reality. We believe that gene editing and cell memory programming are technologies that will drive the future of medicine.
As all biological entities are ultimately defined by their genome, applications may be found in virtually all living systems.
Alzheimer’s disease, diabetes, atherosclerosis, non-toxic therapies, neurological recovery after stroke, regeneration of cardiac tissue after a heart attack
Modulating cell functionality to increase specific outputs
Improved crop yields, adaptation to regional environmental shifts
Coral reef adaptation to warmer ocean temperatures, improved biodiversity